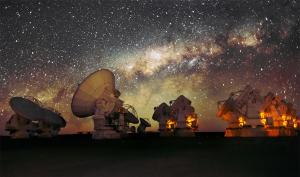
The big secrets of the universe, like how black holes exist in space and time, how planets form and how the universe is evolving, could remain locked away if scientists and commercial users cannot find a way to share the airwaves.
Like detectives with magnifying glasses, astronomers sleuth through space using specialized telescopes that examine radio waves, searching for elements of life and the chemical signatures of star and galaxy formation, and studying difficult-to-detect phenomena such as black holes and star nurseries.
For decades, astronomers have enjoyed unfettered access to the highest, most pristine part of the radio spectrum. That changed when the Federal Communications Commission widened spectrum access to support 5G-and-beyond wireless communications and the billions of connected devices making up the internet of things.
Because radio telescopes are so sensitive and do not tolerate interference, these commercial applications have begun to encroach on scientists’ missions.
Engineers at the University of Virginia are central to the global search for solutions as advances in communication and commercial wireless technologies blur the boundaries that traditionally have separated the users of the electromagnetic spectrum.
You are likely most familiar with your radio dial, which uses the lowest part of the electromagnetic spectrum (called the “radio frequency spectrum”), with a ceiling of 300 megahertz. The next tier is the microwave range, where much of the world’s radars and the Global Positioning System operate. The microwave range starts at 300 megahertz and extends to 30 gigahertz.
The newest smart phones advertise faster speeds through networks that will operate in the next-highest tier of the electromagnetic spectrum — the millimeter wave range. This part of the spectrum extends from 30 to 300 gigahertz and is one key to realizing wide-scale development and deployment of 6G technology. Devices that connect to the internet, process large amounts of data and run complex apps, such as systems that guide autonomous vehicles, will require access to this part of the spectrum.
Different radio telescopes can operate over this entire range of the electromagnetic spectrum and even into the sub-millimeter wave range, which reaches from 300 gigahertz to 30 terahertz. The millimeter and submillimeter range is the part of the spectrum that is needed in particular for scientific endeavors, and where UVA’s part of the story unfolds.
Today, available solutions for high-frequency spectrum sharing include fencing off a portion of the spectrum for certain users, a method called set-asides; scheduling spectrum use; and allowing spectrum owners to make trades.
Robert M. "Bobby" Weikle, professor of electrical and computer engineering at UVA’s School of Engineering and Applied Science, is pursuing a fundamentally different approach to spectrum sharing. He aims to innovate transmitters and receivers so high-sensitivity radio telescopes can ignore competing signals while performing their scientific missions.
“Noise canceling headphones is a very good analogy,” Weikle said. “This is one of the main ideas we are working on. It would require setting sensors around the radio telescope to detect all the signals coming in regardless of source, and then characterize the interference well enough to cancel it out.”
This is a bold idea, because it requires signal processing and sensing capabilities that do not yet exist.
To create these capabilities, Weikle has assembled a research team whose members are recognized internationally for their scientific research on microwave and terahertz devices and signal processing. The team earned a planning grant from the National Science Foundation’s Spectrum Innovation Initiative in August 2020 and subsequently joined with Notre Dame and the University of Colorado to co-found SpectrumX, a $25 million, NSF-funded National Center for Spectrum Innovation launched in September 2021. University of Notre Dame professor of electrical engineering J. Nicholas Laneman leads the center; Weikle chairs the steering committee and leads the working group on radio and network technologies research.
“UVA’s expertise in millimeter and submillimeter science and technology has come full circle with the establishment of SpectrumX,” Weikle said. “We are broadening our focus, from making the most sensitive instruments we can to maintaining sensitivity with tolerance for other users.”
Weikle attributes his team’s leading role in SpectrumX to a decades-long partnership among UVA Engineering, the National Radio Astronomy Observatory and Virginia Diodes, a company founded by UVA electrical engineers and a leading producer of millimeter wave and terahertz test and measurement equipment and components.
The partnership’s groundbreaking achievements have made Charlottesville a nexus for the world’s radio astronomers.
The Beginning of a Beautiful Friendship
The story of why Weikle’s team may be among the best-prepared in the world to rescue radio telescopes from interference began a long time ago at UVA, with a researcher named Robert J. Mattauch.
When Mattauch arrived at the University in 1966 as a new faculty member in the department of electrical engineering, he said, “I was dead set on starting a semiconductor device lab on par with my experience at Carnegie Mellon and Carnegie Tech.”
His vision went against the conventional wisdom of the day, which placed semiconductor research in the physics department. To gin up interest in his first course offering, Mattauch hand-carried a poster across McCormick Road to the physics department, then up to chemistry, then to materials science and engineering. Having attracted the required number of students to enroll, the first microelectronics program at a Virginia university was up and running.
Mattauch earned a small grant from NASA’s Langley Research Center and pieced together the equipment he needed.
“We built a reactive ion etcher out of an old ham radio,” Mattauch recalled. “To produce high ultraviolet intensity, we ‘repurposed’ a big mercury vapor light bulb from a street lamp on McCormick Road, right outside Thornton Hall. We used a Dremel tool to cut off the outside glass and put the bulb’s quartz envelope with its mercury vapor inside a stovepipe. To operate the stovepipe shutter, we used a little kitchen timer with a microwave switch built by one of my grad students.”
Mattauch’s fortunes changed in 1969 when Sandy Weinreb, then head of the electronics division of the National Radio Astronomy Observatory, asked him to help solve a problem with the observatory’s receivers.
“I said to Sandy, ‘If it can be made, I can make it,’” Mattauch said. “Sandy gave me $50,000 and outfitted our lab with all kinds of equipment.”
Mattauch’s and Weinreb’s collaboration produced a long line of Schottky diodes – semiconductor devices named after German physicist Walter H. Schottky – a key technology to detect and measure phenomena through radio astronomy and the study of how matter absorbs and emits light and other radiation.
“Radio astronomers look at molecules in outer space,” Mattauch explained. “When the sun hits the molecule, the whole business, its atoms and bonds, will ring. The frequencies at which these things resonate are essentially thumbprints of their compounds.”
The higher the frequency a radio telescope can detect, the more detailed a look at space phenomena astronomers can get.
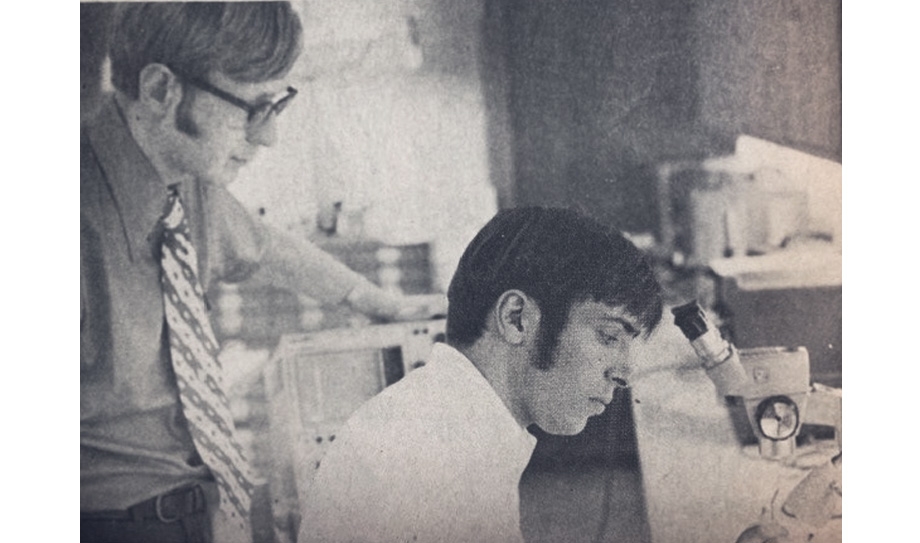
Mattauch and his first Ph.D. student, Tom Viola, hit pay dirt in 1971, fabricating a solid-state device exhibiting the highest sensitivity ever made, with the ability to detect phenomena in the millimeter wave range. By 1973, the devices resulting from Mattauch’s and Weinreb’s joint research were used exclusively in the vast majority of radio telescopes around the world.
“We were the only lab in the world that could make these devices for very high frequency receivers,” Mattauch said. Astronomers from England’s Jodrell Bank Observatory, Germany’s Max Planck Institute and China’s Purple Mountain Observatory made the pilgrimage to Charlottesville to discuss their research needs.
The Successor Generation
Throughout the 1970s, radio astronomers pushed the limits on Mattauch’s semiconductor devices. To detect and understand the composition of complex molecules of outer space, radio telescopes needed to detect higher frequencies, ultimately reaching into the terahertz range.
Mattauch’s pioneering spirit attracted new talent to UVA Engineering. Thomas W. Crowe, president and CEO of Virginia Diodes, Inc., and Arthur W. Lichtenberger, research professor of electrical and computer engineering at UVA, joined Mattauch’s semiconductor lab as graduate students. Each made the laboratory their home as research scientists after they earned their degrees.
Crowe advanced the state-of-the-art of Schottky diodes, designing and deploying diodes that could detect phenomena in the terahertz range, enabling discoveries made by dozens of research groups around the world.
But as radio astronomers expanded their gaze to the furthest reaches of the galaxy, they hit a barrier: Existing receivers were not sufficiently sensitive to detect the faintest of signals with wavelengths measured in millimeters.
“We needed something else,” Mattauch said.
Something else turned out to be someone else – Lichtenberger, who had just begun his graduate studies in physics at UVA.
“I was walking from the physics department to my apartment on Cherry Avenue,” Lichtenberger said. “It was chilly, so I took a shortcut through the Engineering School grounds, and I saw a sign.”
Mattauch had taken advantage of the Engineering School’s annual open house, placing a placard on Engineer’s Way inviting passersby to visit the world-famous semiconductor device laboratory.
“I walked upstairs and Bob Mattauch opened the door. He took one look at me and said, ‘What are you doing here?’”
“I was surprised because Arthur was wearing a T-shirt for the Five College Radio Astronomy Observatory,” Mattauch said.
Mattauch was very familiar with the observatory at the University of Massachusetts, where Lichtenberger had worked after earning his bachelor’s degree at Amherst College.
Their chance meeting revealed they shared many questions about the phenomena that could be revealed through radio astronomy. Lichtenberger decided to transfer to electrical engineering while still standing in Mattauch’s doorway. The next week he did the paperwork, finished out his semester and completed a summer physicist-to-engineer intensive course.
Lichtenberger wanted to seize on the potential of superconducting materials. He told Mattauch about his experiments with low-temperature helium films, using vacuum pumps, and transferring helium in cryogen, all of which are fundamental to the superconducting devices that observatories needed to extend their extragalactic reach.
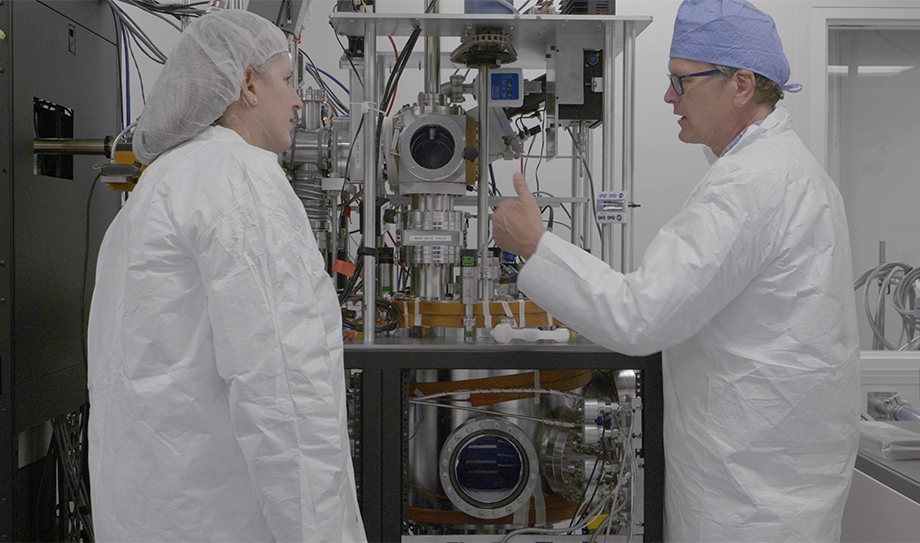
“A receiver is like an ear,” Mattauch said. “You’re listening for something but can’t hear because there is this little gnat in your ear making internal noise. That’s what happens in the semiconductor when electrons bang into each other.”
Superconducting devices, by contrast, can hear very faint signals because they have very low noise.
“Arthur saw that potential for superconductors to look at information from the universe at lower power, and faster,” Mattauch said. “He grabbed onto it and owned it.”
Lichtenberger likens his devices’ capabilities to harmonics. “If you hit two piano notes that are close to each other, you can hear this wavering back and forth, the beat note,” Lichtenberger said. “This happens because the piano body itself is a resonator. It allows two frequencies to interact and mix together to form a different, lower frequency.”
Lichtenberger’s superconducting device works the same way, but electronically. It detects a terahertz signal signature and mixes it with a singular stable frequency tone that is somewhat close, so that the difference output is in the more easily measurable lower gigahertz range. Importantly, it translates all of the information of the original high-frequency signature to the lower frequency band while introducing only an ultra-low amount of noise. This enables astronomers to recognize signatures of organic molecules, as well as signatures from gas clouds and supernovas, and to understand how radiation spins into a black hole.
The reputation of Mattauch’s semiconductor laboratory continued to grow with Crowe’s and Lichtenberger’s advancements in diodes and detectors.
“Pretty much everyone doing work at these high frequencies would go to UVA for devices and technologies they needed,” Weikle said.
Weikle met Mattauch at the California Institute of Technology, which Mattauch visited frequently, and where Weikle earned his master’s and Ph.D. degrees in electrical engineering. Weikle saw an opportunity to expand the UVA laboratory’s capabilities beyond the diodes themselves to designing and building integrated systems and instruments. Weikle joined UVA’s team in 1993 as new faculty member.
Weikle was becoming an expert in how to design and create new millimeter-wave systems using large arrays of coupled devices. He earned the 1993 Microwave Prize, an international award granted by the Microwave Theory and Techniques Society, for this published work, judged the most significant in the field. Millimeter-wave systems were a hot topic for the engineering community at the time, promising to improve on microwave systems’ bandwidth and resolution with new capabilities to penetrate fog, clouds and dust.
Their innovation produced systems that were tunable. Similar to a tuner knob on a radio dial, the team could adjust system parameters such as power level and operating frequency to optimize a receiver array’s reception quality when operating at a specific frequency. Their work was a milestone toward modern imaging and radar systems for transportation, health, weather forecasting and many other daily needs and conveniences.
Tunable millimeter-wave systems for radar captured the attention of the U.S. Army’s National Ground Intelligence Center that is headquartered in Charlottesville. Crowe forged a partnership with the center to provide semiconductors for its advanced radar systems, earning successive five-year contract renewals.
When Crowe and research scientist Bill Bishop co-founded their spin-off company, Virginia Diodes, Inc., in 1996, Weikle naturally assumed leadership of UVA’s partnership with the center. Weikle matured the University’s relationship into the center’s sole provider of specialized submillimeter-wave technology, an agreement most recently renewed in 2021.
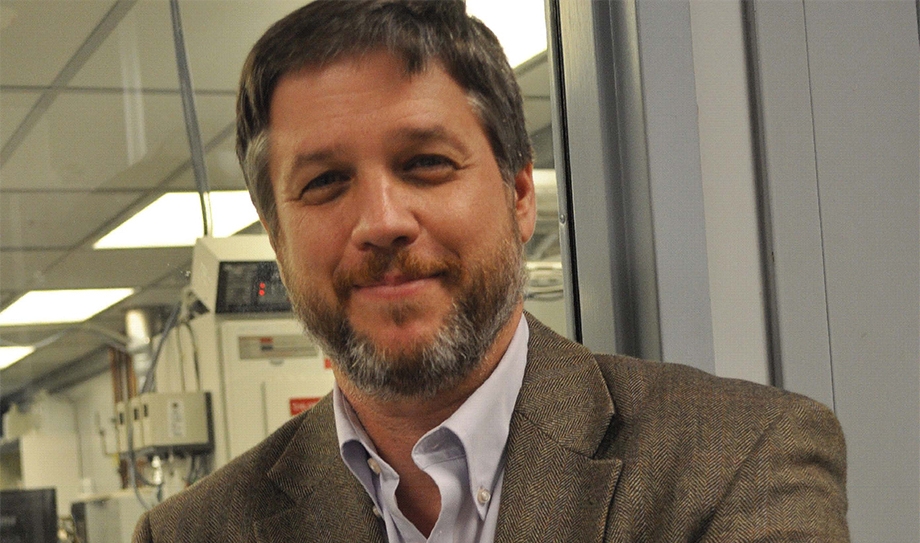
“I see myself as a steward of this unique area of excellence,” Weikle said. “I hope to continue building it into a state-of-the-art strong program for cutting-edge research and technology, and to eventually pass this legacy on to its next proponent, to pick up the banner and keep it going.”
Weikle also helped the semiconductor laboratory enter a completely new arena of testing and evaluation.
“Bob’s and Tom’s work on terahertz diodes, in a sense, really started much of the work on terahertz electronics,” Weikle said. “People who were starting to develop semiconductor electronic devices for extremely high frequencies did not have the tools needed to measure and characterize device performance.”
Standard practice at the time involved melding a chip as thin as a human hair in a fixture with connectors and instruments to measure the semiconductor’s performance. The testing process measured not only the semiconductor but also all the extraneous wiring and interfaces connected to it, which confounded the test results.
Weikle, Lichtenberger and N. Scott Barker, UVA professor of electrical and computer engineering, developed a unique probe that eliminates this superstructure. With diodes embedded into the probe itself, it can measure the performance of high-frequency semiconductors quickly and accurately.
Their probes have proven to be an enabling technology to measure accurately the performance of high-frequency devices, circuits or other components. Anyone working on terahertz technologies can use the probe to characterize their devices to redesign, optimize and improve performance. Because the instruments taking measurements can be integrated into the probe itself, their probe allows developers and manufacturers to conduct routine device testing with a smaller footprint. The integration of instruments into the probe remains an area of active research by their group members; future innovations could lead to cost benefits to make the technology more accessible to others.
From the semiconductor lab’s humble beginnings to the newly renovated Innovations in Fabrication Facility, UVA’s partnerships and spin-offs have produced state-of-the-art superconducting receivers used in radio telescopes throughout the world.
Crowe and his team at Virginia Diodes continue to meet needs for radio astronomy and high-frequency radar.
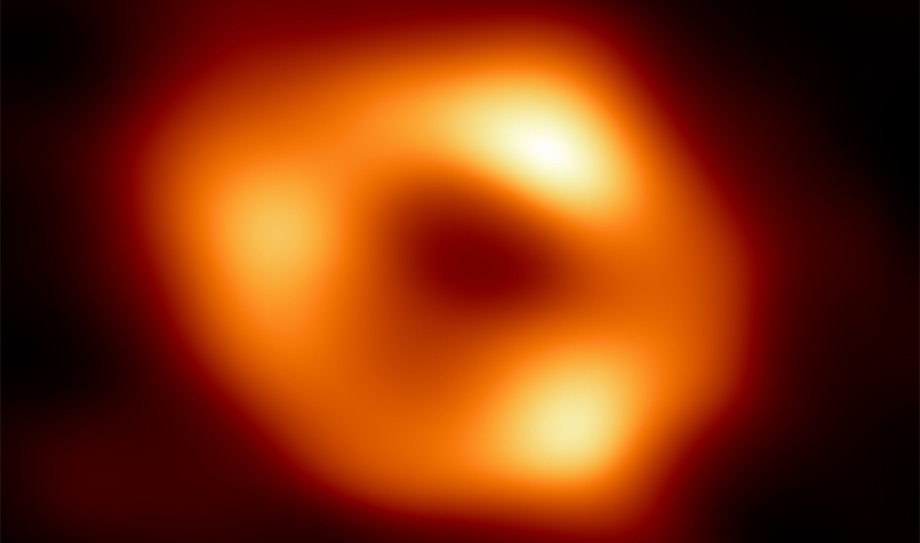
Lichtenberger’s group has built all of the more than 150 specialized detectors for the largest and most sensitive submillimeter radio telescope in the world, the Atacama Large Millimeter/submillimeter Array in Chile, which helped capture the first-ever image of a black hole in 2019. Lichtenberger’s research team is working on wider bandwidth and more sensitive detectors for the Atacama array, supported by fellowship and seed funding from the National Radio Astronomy Observatory as well as direct funding from the international partnership that runs the array.
Weikle, Lichtenberger and Barker have connected UVA’s legacy work with new probes, producing instruments that were not possible 10 to 15 years ago. They co-founded Dominion Microprobes, Inc., in 2011 to design and manufacture probes and associated components for electrical measurement of high-frequency devices and materials.
“If I could sum up Bob’s legacy in one word, it would be curiosity,” Weikle said. “What can I do with this technology? Can I make something that allows me to measure or see something people haven’t been able to do before? To do an experiment, compare it with a model or theory and improve on it.
“This is what engineering is all about: to build new things that improve on what has come before,” Weikle said. “Ultimately, to impact people’s lives in a positive way and use these concepts and technologies to build useful things for humanity.”